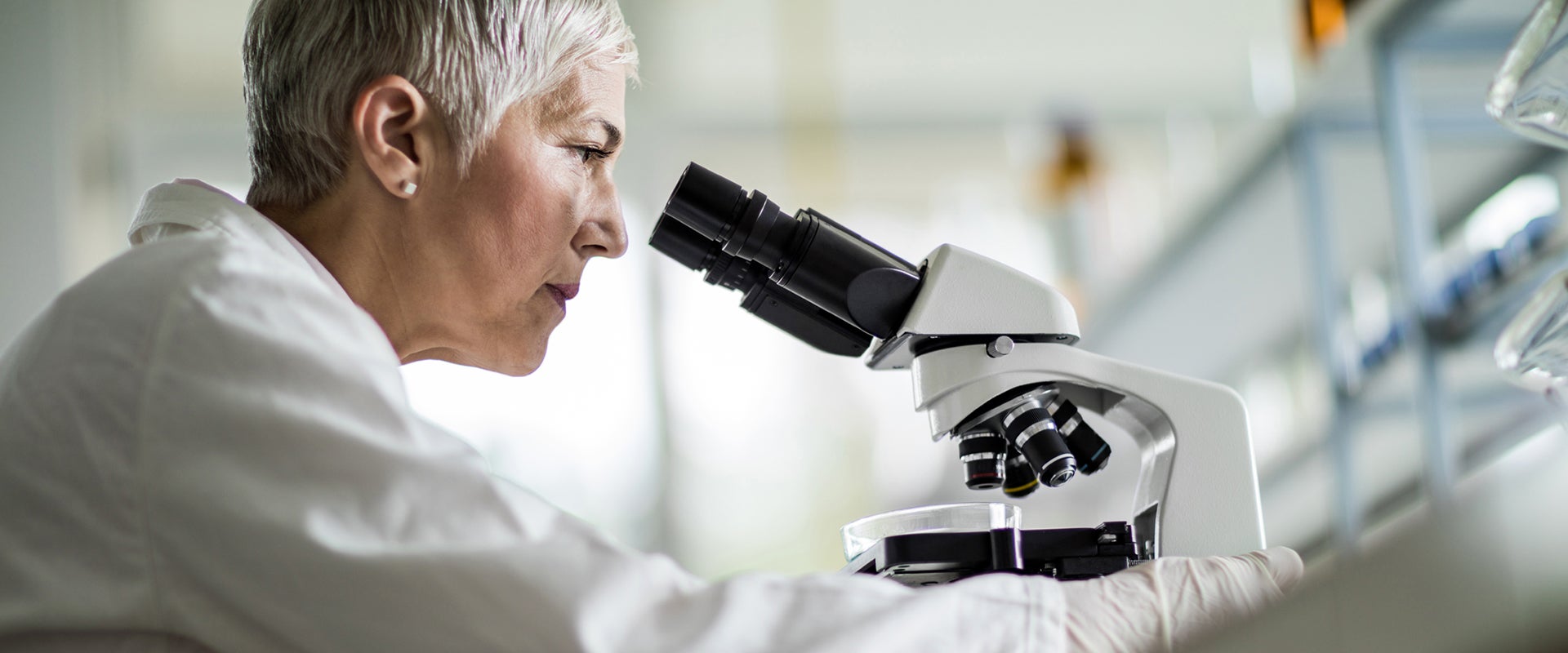
Immuno-Oncology Is Making Pharma Step Up Its Diagnostics Game
- VOLUME XX, ISSUE 28
- Executive Insights
Immuno-oncology represents a paradigm shift in cancer treatment, and its diagnostic needs include identifying patients most likely to respond to therapies.
Current diagnostic approaches often fall short, due in large part to the complexity of the biology driving therapy responsiveness.
The biopharma industry is exploring approaches covering tumor, immune and even microbiome-related pathways and biomarkers.
Just like the immune system, the industry needs to adapt to enable immuno-oncology companion therapies and diagnostics to reach their full potential.
Immuno-oncology has significant diagnostic needs, including identifying patients most likely to respond to therapies. Current diagnostic approaches often fall short, due in large part to the complexity of the biology driving therapy responsiveness. As such, the biopharma industry is exploring many emerging approaches covering tumor, immune and even microbiome-related pathways and biomarkers.
Immuno-oncology represents a paradigm shift in cancer treatment, with the first wave of PD-1/PD-L1 checkpoint inhibitors such as Opdivo (nivolumab, from Bristol-Myers Squibb Co.) and Keytruda (Merck & Co. Inc.’s pembrolizumab) demonstrating cure-like performance in selected metastatic tumors such as nonsmall cell lung cancer (NSCLC) and melanoma.
This efficacy is driving significant checkpoint inhibitor adoption, and analysts project the class alone could represent a $29 billion global market by 2022. In short, checkpoint inhibitors are providing hope to metastatic patients who were previously considered to be on a path to palliative care.
Despite their performance, checkpoint therapies have a number of shortcomings. For instance, response rates are still only 20 to 30% on average (although there is significant variance in published response rates by tumor type), and response times can be prolonged, which can be an issue for patients with advanced metastatic disease.
Checkpoint inhibitors also come with significant side effects, especially when used in the combination regimens (e.g., with anti-CTLA-4) that are ubiquitous in the industry. (Also see “Combinations Continue to Drive Immuno-Oncology DealMaking,” In Vivo, May 2017.) Checkpoint inhibitors’ cost (at up to $150,000 per year for monotherapy) represents a significant burden to healthcare systems and payers. Furthermore, prior exposure to checkpoint therapy may render patients ineligible for other immune-oncology clinical trials.
Today, PD-1/PD-L1 immunohistochemistry tests (IHCs) are available as companion or complementary diagnostics for many approved indications, yet their predictive power is considered limited in many situations. As such, while these IHCs are broadly ordered by clinicians, decisions to use checkpoint therapy may often be based on lack of therapeutic alternatives coupled with substantial patient demand. This is creating significant need for improved diagnostics to predict response to checkpoint therapy, to monitor response and to support sustained usage.
Cancer pathways are already complex, and when you layer in the need to understand the host’s immune system and potentially the microbiome, the complexity is greatly increased. Furthermore, given the inherent heterogeneity of both the tumor and immune cells, understanding the biology may be required down to the single-cell level.
Through a systematic review of clinical trials involving checkpoint inhibitor therapies, we identified over 1,200 ongoing or completed clinical trials involving checkpoint inhibitors going back to 2011. We then mapped the biomarker activity covered in those trial protocols to develop insights on pathways and biomarkers under exploration by biopharma and academic sponsors. Based on this mapping exercise, immuno-oncology biomarkers in solid tumors were broadly categorized into four areas:
Multiple pathways and biomarkers are being explored across categories. Neo-antigen generation trials are looking at TMB (tumor mutational burden), MSI (microsatellite instability) and DNA repair dysfunction. All three rely on the simple premise that the more mutated or genetically unstable a tumor, the more foreign it will look to the immune system. TMB’s role in checkpoint therapy responsiveness emerged quickly from collaborative tumor profiling efforts by academia and clinical laboratories such as Foundation Medicine Inc. and has since garnered significant attention and investment.
Immune activation biomarkers are focused on measuring the presence of cancer-killing immune cells such as CD8+ T cells and the clonal expansion of those T cells, which suggests the immune system has recognized the cancer and is proliferating in preparation for tumor attack. Detection of killer immune cells has traditionally relied on immuno-phenotyping based largely on cell-surface markers, but recent activity also highlights interest in looking at HLA (human leukocyte antigen) genotypes and how they correlate with checkpoint therapy responsiveness. Expression of biomarkers correlated with immune pathway activation such as INF-gamma is also being explored, as they are indicators of immune-mediated killing of cancer cells.
But cancers have cloaking mechanisms, and this drives the need to look at immune-evasion biomarkers, which today focuses primarily on checkpoint activation. The current wave of cancer immunotherapies relies on the disruption of the PD-1/PD-L1 checkpoint interaction, and measuring PD-1 or PD-L1 expression is often correlated with inhibitor response. The industry is also beginning to look into the role of the microbiome in PD-1/ PD-L1 inhibitor therapy responsiveness, and this may represent an important use case in microbiome-based diagnostics.
The sheer growth in trial numbers is impressive, but there is another important trend to call out, which is the number of unique biological pathways/biomarkers being assessed per trial (see Figure 2).
Since 2015, there has been notable growth in trials measuring two or more pathways as part of the trial design, and this trend appears to be accelerating, with some trials looking at four or more pathways. It is important to note that while many trials may not specify a biomarker, many require tumor biospecimens as an enrollment criterion, suggesting that biomarkers may be explored and even submitted to regulators regardless of whether specified in the trial protocol. It is also important to mention that merely highlighting interest in exploring a particular pathway or biomarker as part of a clinical trial does not necessarily lock in a biomarker as part of the drug label.
Checkpoint activation biomarkers (PD-1/PD-L1) have seen the most activity and sustained growth, as they are directly related to the mechanism of action of checkpoint inhibitor therapies. However, exploration of other pathways and biomarkers is occurring in parallel. Trials looking at immune activation biomarkers, including immune stimulation, immunophenotyping (this includes tumor infiltrating lymphocyte [TIL] counting) and TCR (T-cell receptor) clonality, have exploded since 2015 and are now being explored in 40% of biomarker-specified trials initiated in 2017.
Neo-antigen generation biomarkers have also grown significantly since 2015, with emphasis on MSI and TMB. MSI (for which Merck & Co. gained the industry’s first biomarker-defined drug label) activity is the highest of the two, but it is also a more established biomarker, whereas TMB, which was only discovered in 2015, is already being explored in registration trials by a number of biopharma players, including Roche and Bristol-Myers Squibb. (Also see “Bristol’s Opdivo/Yervoy Bid Will Show Whether Tumor Mutation Burden Is Ready for Prime Time,” Pink Sheet, February 5, 2018.)
Since 2015, there has also been an uptick in assessing non-checkpoint regulatory biomarkers, such as IDO and FOXP3 (representing 8% of clinical trials initiated in 2017). And although still small today, there is a clear interest in assessing the microbiome’s role in checkpoint therapy trials (< 3% of trials initiated in 2017). (See Figure 3.)
Looking at biomarkers by development phase highlights that while checkpoint activation biomarkers are the most represented pathway in Phase III trials, most other pathways are being explored across development phases (see Figure 4).
Not surprisingly, overall trial activity is highest in NSCLC and melanoma, as these are indications where the first checkpoint therapy approvals were awarded. But there is also meaningful trial activity in other solid tumors such as breast, head-and-neck, renal, colorectal (CRC), bladder and even liquid tumors. Interestingly, biomarkers are being explored across all tumor types, with CRC over-indexing relative to other tumors (due to the well-established link between MSI in CRC), whereas HCC (hepatocellular carcinoma), pancreatic and gastric cancers are under-indexing on a relative basis.
Ultimately, with few exceptions, it appears that most tumors will benefit from biomarker analysis to predict checkpoint inhibitor responsiveness. That said, the industry needs to go through this current R&D wave to understand which biomarker strategies work best by tumor type and indication (see Figure 5).
A possible future outcome may be that many pathways and biomarkers will need to be assessed regardless of tumor type, to tailor immuno-oncology therapy to patients by looking at the underlying biology of the tumor, the host immune system and even the microbiome.
Given the number and types of biomarkers explored (DNA, RNA, protein), multiple diagnostic modalities are being employed, including next-generation sequencing (NGS), quantitative polymerase chain reaction (qPCR), NanoString Technologies Inc.’s nCounter, IHC and flow cytometry. NGS (covering both DNA and RNA sequencing) appears to be poised to address the majority of immuno-oncology biology and pathways, and its increasing prominence in tumor profiling in metastatic disease could position the technology as a front-runner in immuno-oncology diagnostics. However, high-parameter flow cytometry and IHC are also expected to be important immuno-oncology diagnostic tools in the long run given their ability to detect expressed proteins at the single-cell level (see Figure 6).
Another notable point in the immuno-oncology diagnostics space is the emergence of multiparameter RNA expression and single-cell NGS. Multiparameter expression analysis has the potential to include multiple areas of immuno-oncology biology and pathways, essentially covering both immune activation and evasion. And because both tumors and the immune system are marked by their cellular heterogeneity (due to the genetic instability of tumors and the adaptability of immune cells to new threats), the need for single-cell NGS is also gaining prominence in research.
Although the cost to assess multiple biomarkers to predict checkpoint responsiveness may represent a step-change from the single biomarker companion diagnostics associated with most targeted therapies, the clinical and economic needs associated with tailoring the use of checkpoint inhibitors will likely support this added cost.
It is important to note that many diagnostic enablers, including platform companies such as Illumina Inc., Thermo Fisher Scientific Inc., HTG Molecular Diagnostics Inc. and NanoString, as well as clinical laboratories such as Foundation Medicine, OmniSeq LLC and Caris Life Sciences, are developing immuno-oncology diagnostic solutions that span multiple biological pathways and are actively partnering with pharma (see Figure 7). OmniSeq, a clinical laboratory associated with Roswell Park Cancer Institute, now offers Immune Report Card, which looks at pathways across neo-antigen generation, immune activation and immune evasion. A recent commercial partnership between OmniSeq and Laboratory Corp. of America Holdings has the potential to significantly expand access to OmniSeq’s Immune Report Card, leveraging LabCorp’s extensive channel.
Against this backdrop, there is an acceleration in the biomarker innovation cycle driven by the comprehensive genomic profiling of tumors using NGS. L.E.K. Consulting estimates that 10-20% of metastatic patients receive NGS profiling in the U.S. today, and that number could increase two to three times in the next three to five years (which represents hundreds of thousands of patient cases annually).
Many of the NGS providers are building large data sets incorporating genomics and clinical and outcomes data, which is enabling identification and retrospective validation of new biomarker associations directly from real-world clinical cases. This activity has the potential to validate new biomarker associations backed by a significant number of patient cases (often dwarfing what is feasible with traditional clinical trials). Supported by this strength of evidence, it is expected that guidelines and clinical adoption of these biomarkers will follow quickly. Conceptually, this represents the application of big data in precision genomic medicine.
However, as highlighted earlier, this is no longer purely conceptual. The rapid rise of TMB represents a paradigm shift. TMB isn’t even a definable genomic biomarker in the traditional sense. TMB is not affiliated with a specific gene or pathway, but rather it is an observation that tumors with a relatively high frequency of mutations (high TMB) tend to respond better to checkpoint inhibitors (thought to be due to increased neo-antigen presentation). Interestingly, TMB also represents a new situation where diagnostics companies have forged the path and biopharma companies have followed suit. This stands in significant contrast to other targeted therapies where biomarkers and their associated complementary/companion diagnostics were largely validated by biopharma-sponsored pivotal trials.
TMB is also creating a virtuous discovery cycle that may only continue churning out new and more nuanced biomarker associations. NGS is required to measure TMB, and as TMB becomes more routinely adopted, it is an important driver to continued NGS adoption. Furthermore, NGS panel sizes continue to grow, and digitization of healthcare data by players such as Flatiron Health Inc. and COTA Inc. is enabling the creation of massive minable data sets.
There is a long road ahead for the immuno-oncology diagnostics space to mature. As discussed earlier, the science and supporting evidence need to be developed, and there are many directions in which they could go. Will it end with a reductionist biomarker strategy reliant on a few highly predictive biomarkers, or will it end with a more comprehensive biomarker strategy that looks at many pathways in concert? Certainly, the biology of immuno-oncology therapies, which relies on the interaction between a dynamic tumor and the immune system, suggests the more comprehensive strategy may prevail in the long run, but it will take time for that to materialize.
Separately, the industry needs to drive standardization, not only in pathways and biomarkers assessed, but also in defining standards around interpretation, including defining thresholds for what constitutes a biomarker-high or biomarker-positive result. Standardization and concordance across sample types assessed will also need to be worked out, including understanding cell heterogeneity (for both tumor and immune cells) and between tissue-based samples and those derived from biofluids, including CTCs, peripheral immune cells, cell-free components, exosomes and so forth.
The industry will also ultimately want to consolidate testing into a standard set and will not support a different test for every therapeutic option under consideration. This means there will need to be cooperation and collaboration across the industry, and looking at other diagnostics markets, a reasonable assumption suggests three to five major competitors will emerge as leaders. Access to novel diagnostic approaches will also need to be addressed. Today, many of the emerging immuno-oncology diagnostic tests require specialized instrumentation and operators, may take many weeks to process and are not well-reimbursed. Clearly, as the industry scales, this will need to change and fit better into therapeutic decision-making windows and healthcare economics.
Biopharmaceutical companies will need to adapt in this new high-paced biomarker environment. Trying to stay abreast of the biomarker landscape in a sequential fashion with each new biomarker approval may put companies into endless catch-up mode.
An alternative strategy could be to proactively assess multiple pathways and biomarkers at the outset, leveraging basket trials with fast conditional approvals to match therapeutic options to each patient’s unique (and ever-changing) biomarkers.
Organizationally, biopharmaceutical companies will need to change across many functions. Deeper embedding of biomarker/ diagnostics groups into development and commercial functions will be required. Business development activity with diagnostics partners will need to focus on broader collaborations with a focused set of diagnostic companies, with the ultimate goal of creating industry-standard diagnostic solutions. Market access and pricing functions will also need to consider how evolutionary biomarker strategies impact pricing and reimbursement of both the immuno-oncology diagnostics and therapeutics. Regulators will do well to enable such forward-thinking approaches. Just like the immune system, the industry needs to adapt to enable immuno-oncology companion therapies and diagnostics to reach their full potential.
Editor’s note: This article first appeared in In Vivo.